Biocompatibility
How to Apply Extractables and Leachables to Medical Devices
Recent changes to ISO 10993-1 have changed the landscape of medical device biological safety testing. The toxicity test box ticking from the biocompatibility test matrix is no longer used. Materials analysis, chemical risk analysis, analytical chemistry, and toxicological risk analysis have all been introduced.
ISO 10993-1:2018 is the name of the standard (which is not new). Medical device biological evaluation Part 1: Evaluation and testing within a risk management process is meant to reflect the document’s philosophy. It instructs us to assess the risks and manage them. Every device now requires chemical knowledge, according to the new edition. It also instructed the driver to apply this knowledge to determining the device’s potential toxicity. The potential for toxicity then becomes the driving force behind risk assessment, which may eventually lead to the need for testing.
The idea is to list of all your known materials, as well as any known toxicity data. You can then use this information to create a risk assessment for these materials, making sure to include any potential contaminants as well as the device’s function. If your product is non-invasive, has a short contact time, and is made of medically or food-grade materials, you may decide that no additional work is required. The need to chemically characterize the final product grows as the potential harm to the patient grows (due to increased invasiveness and/or longer contact). ISO 10993 part 18 and extractables and leachables become important at this point. If no other sources of information are available, traditional in-vivo studies for toxicity end points must be used.
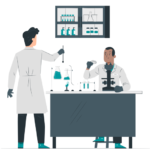
Device Type and Biocompatibility Matrix
The ISO 10993-1 biocompatibility matrix now serves as a tool for determining which information requirements to use. Chemical analysis is now available in all categories. This does not always necessitate testing, but it does necessitate knowledge of potential toxicity.
Device Categories – Definitions & Examples
When using the biocompatibility matrix philosophy, it is clear that the rigor of chemical analysis and any associated toxicological risk analysis can be tailored to the device’s application.
As a result, continuous skin contact would necessitate less intensive extraction and investigation of chemical migrants. A long-term implant, on the other hand, would necessitate extensive extraction and analysis, as well as a thorough risk assessment of all materials identified.
Material Characterisation - Extractables and Leachables
ISO 10993-18
The chemical materials present, as well as the morphology and nature of the surfaces, are all considered during material characterisation as described in ISO 10993-18. Surface features that encourage ingrowth or bacterial colonization may be the focus of the surface investigation. Furthermore, there could be issues with the surface’s chemistry or catalytic properties. Electron microscopy, elemental analysis, infrared spectroscopy, and other techniques could be used in the investigation.
The primary focus of the research will always be on the materials released by the medical device in use. This is the same concept that was used in traditional testing, which used device extracts in in-vivo testing. This is known as an extractables and leachables study in chemical analysis. The requirement for assessing materials migrating from a pharmaceutical container is fairly well defined; however, for medical devices, we must return to the standards and risk analyses.
ISO 10993-17
ISO 10993-17 defines leachables as “released constituents that may come into contact with an individual during clinical use.” The ISO 10993-17 definition of ‘constituents that can be extracted in the laboratory’ includes additional entities that can be forced out of construction materials. In pharmaceutical container studies, the reason for identifying and quantifying the extractables is that there is a risk of them transferring into the formulation at some point during storage. Similarly, extractables in medical devices are being investigated because they may become leachable at some point during the device’s lifetime.
When planning a study, keep in mind that the manufacturing process may involve multiple chemicals (mould release, processing and machinery oils, solvents, UV, or other adhesives). Many of the specified materials (especially plastics and adhesives) may also contain undeclared additives such as activators/accelerators, catalysts, colours or enhancers, lubricants, scratch protection, side reaction products, residual monomers, UV, or other stabilizers. The list is extensive.
The concept of leachables works well in medical devices. Only dry skin is in contact with the adhesive on ECG electrodes. Only materials that could transfer to the skin over a few days are considered “in use leachables.” The patient is given a new dose of leachables if the electrode is replaced after three days. Forcing extractables from the electrode isn’t a good idea in this case. However, there is a risk of chemicals that are more difficult to extract migrating into the patient with a long-term cerebral implant. The concept of “simulated use” leachables is introduced in this section. Clearly, waiting many years for the extractable to migrate into solution for analysis is not an option.
ISO 10993-12
ISO 10993-12 provides us with the extraction conditions (area to volume ratio, time and temperature, solvent polarity), but may not go far enough for more invasive devices.
As a result, forced extraction is used, with the strength of which (while based on ISO 10993-12) can be adjusted depending on the environment and usage duration. Hence the term “simulated use extract.” We increase the solvent strength and consider increasing extraction times and temperatures as we progress up the invasiveness scale. Because we’re only interested in materials that will be used, not degradation products produced during the extraction process, keep this in mind.
Selection of Extractable and Leachable Analysis According to Patient Contact
Following the preceding analysis, it is logical to assign different levels of analysis to meet the biocompatibility matrix’s requirements. (The analytical methods are briefly described at the end of this document.)
Table 1 – Standard surface areas and extract liquid volumes
Table 2 – Standard extraction conditions
Biocompatibility Planning
Device companies spend a tremendous amount of time, money and energy developing and implementing biocompatibility testing programs. Below is a Biocompatibility Planning Tool to guide you through the basic concepts of device testing and to help manufacturers select testing procedures to comply with current regulatory requirements.
The chart below gives you an overview of the process. The potential toxicity of the materials, both individually and in combination, is then quantified using a variety of data sources.
Extraction Media for Biocompatibility Testing
Medical device biocompatibility problems are most often caused by toxins that leach out of the device into the surrounding tissues or body fluids. So, in the laboratory, extracts of device materials are often used in assessing biocompatibility. These extracts are generally prepared using exaggerated conditions of time and temperature to allow a margin of safety over normal physiological conditions.
Analytical extraction studies allow the chemist to identify and quantitate specific leachable moieties. This data can in turn help the device toxicologist or risk assessor determine the worst case scenario for patient exposure and the risk to patient health.
Extracts are also used in many of the biological tests specified by ISO 10993.
Table 1 below lists the most commonly used extracting media. For most devices, only saline and vegetable oil extracts are needed.
Extracts are selected on the basis of the biological environment in which the test material is to be used. A saline (SCI) extract approximates the aqueous, hydrophilic fluids in the body. It also permits the use of extreme temperatures in preparing the extracts, thus simulating certain sterilization conditions.
Tissue culture media may even more closely approximate aqueous body fluids but cannot be used for high temperature extractions. Vegetable oils are non-polar, hydrophobic solvents and simulate the lipid fluids in the body. For technical reasons, DMSO extracts are often used in certain genotoxicity and sensitization tests. Two other common extracting media – Alcohol in SCI and PEG – should be used only if they approximate the solvent properties of drugs or other materials that will contact the device during its normal use.
Extraction conditions (temperature and time) should be at least as extreme as any conditions the device or material will encounter during sterilization or clinical use. Generally, you will want to choose the highest extraction temperature that does not melt or fuse the material or cause chemical changes. To provide some margin of safety for use conditions, Pacific BioLabs recommends an extraction condition of at least 50°C for 72 hours. For devices that are susceptible to heat, an extraction condition of 37°C for 72 hours may be acceptable. Table 2 above lists common extraction conditions.
Sample Preparation and Surface Area Calculation
The simplest method for determining the surface area of a device is usually to use the CAD program from the design engineering group. Typically, the surface area can be calculated with just a few keystrokes. Alternatively, you can calculate the surface area using the equations below. Or you can submit a sample device and/or an engineering drawing to CLIN-r+, and our staff will perform the calculations.
Typically, the standard surface area of your device is used to determine the volume of extract needed for each test performed. This area includes the combined area of both sides of the device but excludes indeterminate surface irregularities. If the surface area cannot be determined due to the configuration of the device, a mass/volume of extracting fluid can be used. In either case, the device is cut into small pieces before extraction to enhance exposure to the extracting media. In some cases, it is not appropriate to cut the device; such devices are tested intact.
The Test Turnaround Time and Sample Requirements table lists the amount of sample required for many procedures. Generally, we recommend using the ratio of sample to extracting media specified in ISO 19993-12 (i.e. either 6 cm²/mL or 3 cm²/mL, depending on the thickness of the test material). For some types of materials, the ratio used for USP Elastomeric Closures for Injections (1.25 cm² per mL) is preferred.
Formulas for Surface Area Calculation
Toxicological Risk Analysis (TRA)
Analytical chemistry provides data on which materials are present and in what amounts. To be useful, this data must be interpreted in terms of the biocompatibility matrix’s toxicity end points. This can be a simple assessment if no materials of concern are found, or the patient contact is brief. The need for a Toxicological Risk Analysis grows as more materials are identified and patient contact becomes more intense. A Registered Toxicologist is responsible for this analysis. Who calculates the patient dose per 24 hours and over the product lifetime for each material discovered? The potential toxicity of the materials, both individually and in combination, is then quantified using a variety of data sources.
A Brief Description of Analytical Methods
LC-MS
Liquid chromatography – At normal temperatures, mass spectroscopy is used to analyze liquid materials. These materials are extracted from a device or container using solvents. The migrated materials’ solvent is then injected into a tube containing a separation media, which separates the chemicals (this works just like blotting paper and ink). The materials are then individually presented to the mass spectrometer for mass analysis. The spectrometer uses technology similar to that of an old television with a cathode ray tube. The electrons in the tube break down the molecules, which are then accelerated towards a target. The time it takes to arrive is proportional to the molecule’s mass and can be used to identify the substance.
MET also has a variant of this machine called an LC-TOF. This Time-of-Flight instrument is extremely accurate, allowing for the identification of unknown materials by revealing their exact masses.
GC-MS
Gas chromatography – mass spectroscopy. This technology is based on the same principles as the LC. At normal temperatures, it examines materials that are gaseous or volatile.
GC-MS/HS
This instrument is a GC-MS with a heating system at the chromatography tube’s entrance point. This enables it to analyse materials with a lower boiling point (higher volatility) than those typically found in gas chromatography and liquid chromatography systems. It’s known as a Headspace GC-MS.
GFAAF
Graphite Furnace Atomic Absorption. This instrument is a lot of fun to play. It works in the same way that salt on a gas stove flame does. The colour of the flame will change from blue to orange. The orange colour (or rather, its wavelength) is specific to the sodium in the salt. This method can be used to identify and quantify each metal’s unique colour range. This is how we learn about star composition, planetary atmospheres, and interstellar gases. The wavelength of light is stretched as stars move away from us, making it redder than we would expect for the ‘metals’ present. This ‘red shift’ tells us how fast the stars are moving.
ICP-MS
Atomic absorption is similar to this analysis. The equipment is less sensitive, but it is more capable of detecting multiple metals.
Analytical chemistry provides data on which materials are present and in what amounts. To be useful, this data must be interpreted in terms of the biocompatibility matrix’s toxicity end points. This can be a simple assessment if no materials of concern are found, or the patient contact is brief. The need for a Toxicological Risk Analysis grows as more materials are identified and patient contact becomes more intense. A Registered Toxicologist is responsible for this analysis. Who calculates the patient dose per 24 hours and over the product lifetime for each material discovered? The potential toxicity of the materials, both individually and in combination, is then quantified using a variety of data sources.
CLIN-r+ top tips on being compliant:
ISO 10993 now requires knowledge of any chemicals released by a device while it is in use. This is now included in the testing matrix for each device category. Although materials characterisation isn’t the only way to get this information, it’s the most likely way to discover unexpected materials. The rigor with which chemical analysis is applied should be tailored to the device’s body contact and risk assessment. Through a Toxicological Risk Analysis (TRA), chemical information can often address most of the toxicity end points without the need for animal testing.
Not all testing labs are created equal, and your auditor will look at this. Ensure the labs you’re using have ISO 17025 accreditation and GLP. It’s also essential that they have a Toxicologist who will compile your Biocompatibility plan and will undersign the TRA and BER.
We hope this document has answered your questions. Should you have any other questions or need professional assistance, CLIN-r+ can be a first point of contact to assess if your device needs biocompatibility testing and suitable labs. Get in touch!
References
- ISO 10993-1:2018: Biological evaluation of medical devices – Part 1: Evaluation and testing within a risk management process
- ISO 10993-18:2005: Biological evaluation of medical devices – Part 18: Chemical characterization of materials3. ISO 10993-17:2002: Biological evaluation of medical devices – Part 17: Establishment of allowable limits for leachable substances
- ISO 10993-12:2012: Biological evaluation of medical devices – Part 12: Sample preparation and reference materials
- ISO 21726:2019: Biological evaluation of medical devices – Application of the threshold of toxicological concern (TTC) for assessing biocompatibility of medical device constituents